Nuclear-powered carbon management options evaluated in DOE report
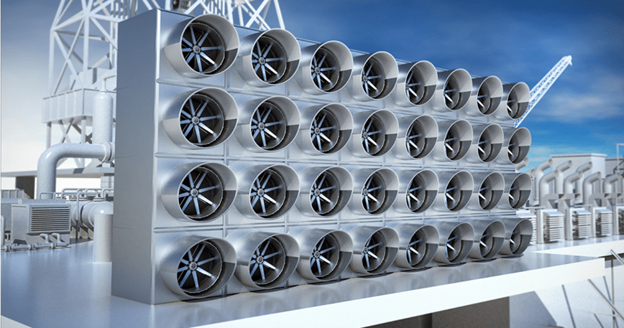
Given how much carbon dioxide has been released into the atmosphere from fossil fuels, replacing those fuels with clean options like nuclear energy is urgent, but could be likened to shutting the barn door after the proverbial horse has bolted. But what if you could also round up excess CO2 already in the atmosphere? That’s the goal of direct air capture (DAC) and other so-called negative emission technologies—to capture climate warming CO2 for use in products or processes or for permanent storage.
The Department of Energy released a report on September 7—Assessment of Nuclear Energy to Support Negative Emission Technologies—that includes a techno-economic study of nuclear-powered DAC systems. Using nuclear power could reduce the levelized cost of solid, sorbent-based DAC systems (S-DAC) by about 13 percent and liquid, solvent based DAC systems (L-DAC) by about 7 percent, compared with the costs for nonnuclear DAC systems, according to the report.
“This report developed with my colleagues from several national laboratories shows that nuclear energy can make the direct air capture process more affordable to remove carbon dioxide from the atmosphere,” said Nicolas Stauff, group manager for nuclear applications and economics at Argonne National Laboratory and lead author on the report. “Such new applications would extend market opportunities for nuclear beyond electricity, transportation, and other industrial applications while increasing nuclear power’s role in the fight against climate change.”
The report represents an “initial exploration” of the role of nuclear power in negative emissions technologies, according to the DOE. It was organized under the Office of Nuclear Energy’s Systems Analysis & Integration campaign, with contributions from researchers from Argonne, Idaho National Laboratory, and the National Energy Technology Laboratory.
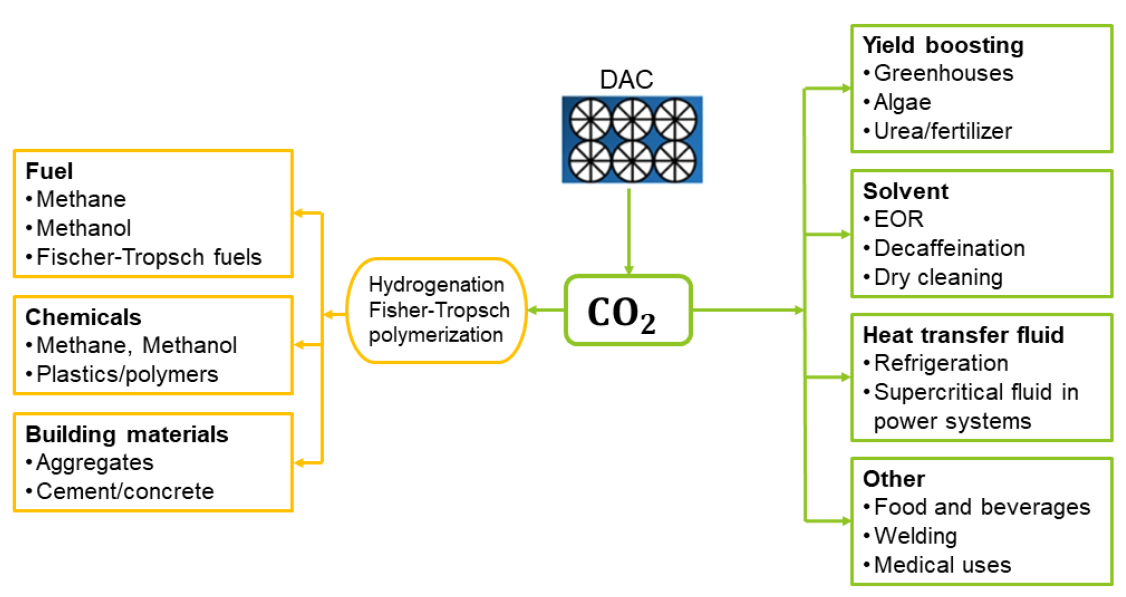
As illustrated in Figure B-1 from the report, captured CO2 could be used as a component of industrial products (left) or could be used directly as a CO2 stream for chemical processes (right). (Graphic: DOE SAIC, Assessment of Nuclear Energy to Support Negative Emission Technologies)
The premise: The DOE estimates that reaching the Biden administration’s goal of net-zero emissions by 2050 will require the removal of between 400 million and 1.8 billion metric tons of CO2 from the atmosphere each year.
DAC is one of the most mature carbon-removal technologies, but it is an energy-intensive (and thus costly) process that requires heat, electricity, and chemical processing of the captured CO2. The systems need electricity to power fans, pumps, compressors, water cooling systems, and air separation units, and heat to drive the chemical reactions that regenerate the solvent or sorbent and concentrate the CO2.
Leveraging carbon-free nuclear electricity and heat would allow DAC systems to be smaller and less expensive to operate since their emissions footprint would be smaller, compared with traditional systems powered by natural gas or the electric grid. Nonnuclear DAC systems need capacity to handle the emissions of their power sources, as well as any captured atmospheric CO2.
The DOE’s Office of Fossil Energy and Carbon Management has already awarded funding for DAC front-end engineering design studies at two nuclear power plants: Constellation’s Byron (L-DAC) and Southern Company’s Farley (S-DAC).
Solid or liquid? It matters: Researchers analyzed S-DAC systems paired with nuclear heat and electricity, and L-DAC systems paired with a combination of natural gas heat and nuclear electricity.
The new study analyzed the potential performance of both systems with three reactor types—a pressurized water reactor (Westinghouse’s AP1000), a sodium-cooled fast reactor (AFR-100, an Argonne-developed design), and a very high temperature reactor (HolosGen’s helium-cooled microreactor)—and showed that both types of DAC systems could use the electricity output of any of the three reactor types. Those reactors can provide direct heat utilization at 273°C, 500°C, and 850°C, respectively—or, if the heat is first used to produce electricity, lower temperature or waste heat could be extracted for use with DAC.
While S-DAC systems would benefit from reactors’ low-temperature heat, “with future technological improvements,” L-DAC systems “could benefit from the high-temperature heat provided by sodium-cooled fast reactors and very high temperature reactors,” minimizing or eliminating the need for natural gas heat.
The researchers found that L-DAC can capture more carbon—a 1-GWt nuclear power plant coupled with L-DAC could capture 12–15 metric tons of CO2 per year, while S-DAC could capture 1.0–1.5 metric tons CO2 per year. While a nuclear-paired L-DAC would reduce costs, compared with a natural gas L-DAC, by about 7 percent (for S-DAC, a 13 percent reduction), L-DAC systems have a lower levelized cost, making it more attractive than S-DAC to markets. Both the solid and liquid approaches represent a “large investment” that “would require long-term certainty of sufficient market size, CO2 prices, and incentives.”
The study concludes “that for both the L-DAC and S-DAC systems, [a nuclear power plant] adds a small economic benefit” when compared to fossil powered DAC system, and a main driver of that benefit is avoiding the cost of fuel for a gas-powered DAC system.
What about other options? Given the very low concentration of CO2 in air (about 400 ppm), DAC requires significant energy input, leading to high costs. The researchers also found potential benefits for using nuclear energy with negative emission technologies other than DAC, such as biomass-based and water-based processes. These technologies may be more cost-effective but are currently at a lower technology readiness level than DAC.
Nuclear energy could provide heat and electricity for biomass combustion, gasification, or pyrolysis—in each of those processes carbon is captured from flue gas, and the biomass combustion process can also produce electricity. Water-based carbon capture could use nuclear electricity and heat for “engineered enhanced weathering,” a process that reacts atmospheric CO2 with rocks for storage as bicarbonate in the ocean. Another process, indirect seawater capture, can convert bicarbonate from seawater into CO2 for geological sequestration.
According to the report, both biomass-based and water-based processes “might use less energy than DAC, since they process carbon that is more concentrated than in the atmosphere, increasing the CO2 stored per NPP, and in some designs they can create valuable byproducts like hydrogen and bio-oil.”