Small and Advanced Reactors with Diverse Fuel Cycles Can Deliver Energy Security
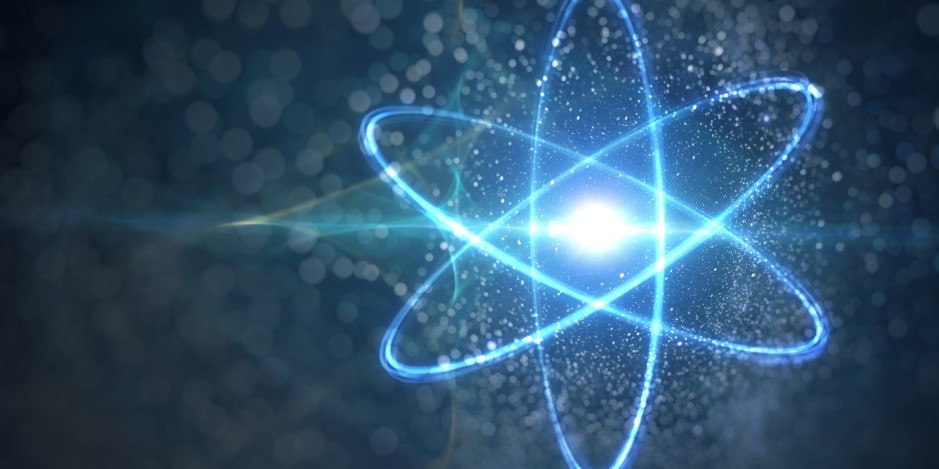
Nuclear energy offers a pairing of high capacity factors and low carbon emissions unrivaled by other power sources, and advanced reactors and small modular reactors (SMRs) hold promise to deliver that power with even more efficiency and versatility. Researchers in the public and private sectors, backed by government funding through programs like the Department of Energy’s Advanced Reactor Demonstration Program and Advanced Research Projects Agency–Energy (ARPA-E), are bringing that promise closer to fulfillment by engineering solutions to open questions about advanced reactor materials and fuel cycles.