The Hanford B Reactor
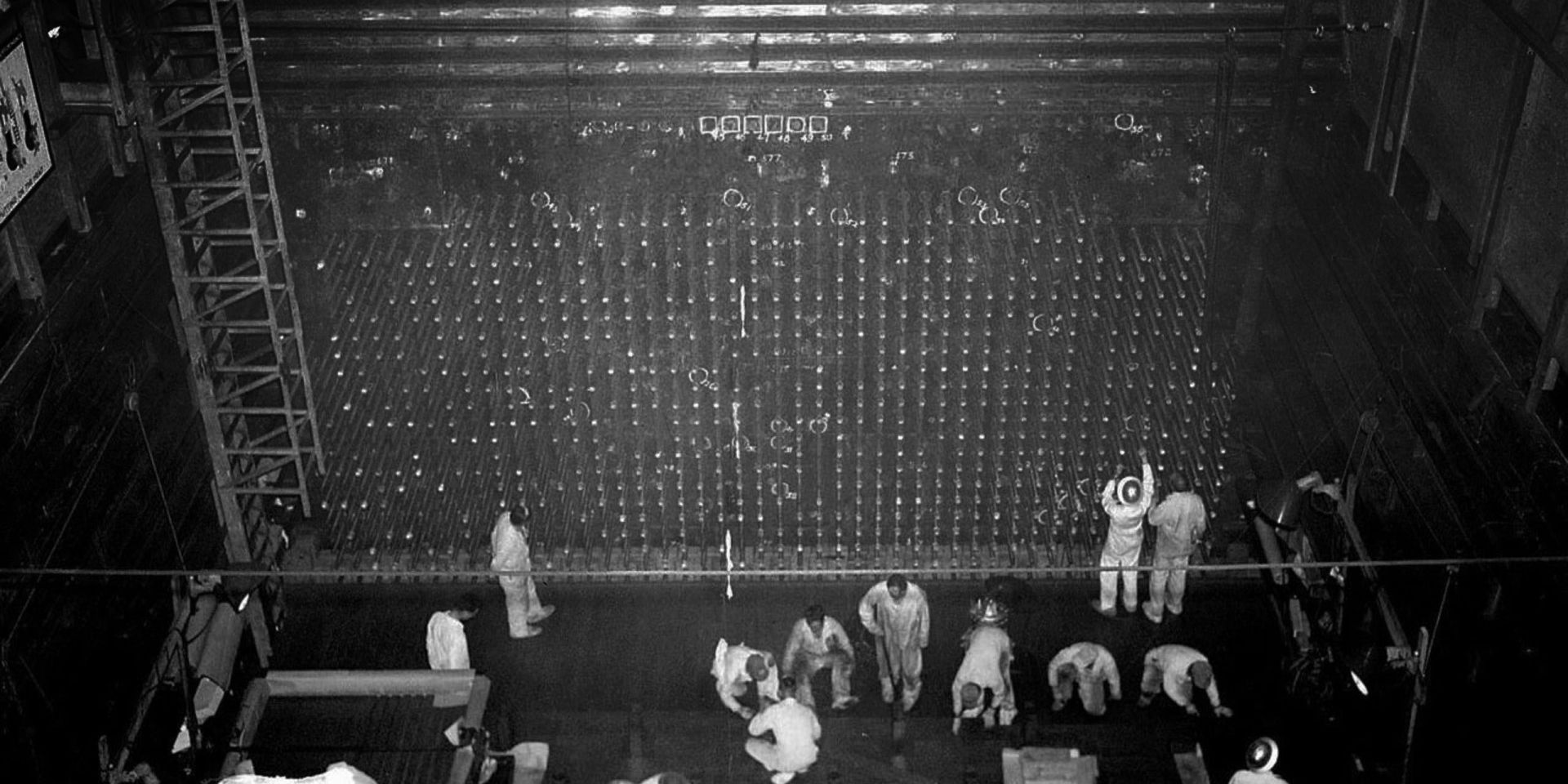
In remote southeastern Washington you will find the sprawling Hanford Site, which was constructed to produce plutonium for the Manhattan Project. Within this complex is the first plutonium production reactor, the Hanford B Reactor. The DuPont Corporation was responsible for construction and operation of the B Reactor. Due to the urgency of the Manhattan Project, construction was completed in just over a year, and The B Reactor went critical on September 26, 1944. After the needs of the Manhattan Project were satisfied, the reactor was briefly shut down and then restarted to produce plutonium for roughly another 20 years, supporting Cold War efforts. In addition to plutonium production, the B Reactor also pioneered the process to produce tritium for the first-ever thermonuclear test.