One year later: Three peer-reviewed papers tell the story of NIF’s record yield shot
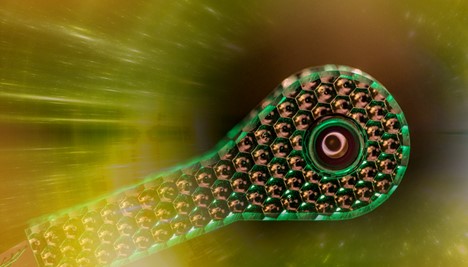
Just over one year ago, on August 8, 2021, researchers achieved a yield of more than 1.3 megajoules (MJ) at Lawrence Livermore National Laboratory’s National Ignition Facility (NIF) for the first time, achieving scientific ignition and getting closer to fusion gain.
The scientific results of the historic experiment were published exactly one year later in three peer-reviewed papers: one in Physical Review Letters and two (an experimental paper and a design paper) in Physical Review E. In recognition of the many individuals who worked over decades to enable the ignition milestone, more than 1,000 authors are included on the Physical Review Letters paper.