Argonne-led team models fluid dynamics of entire SMR core
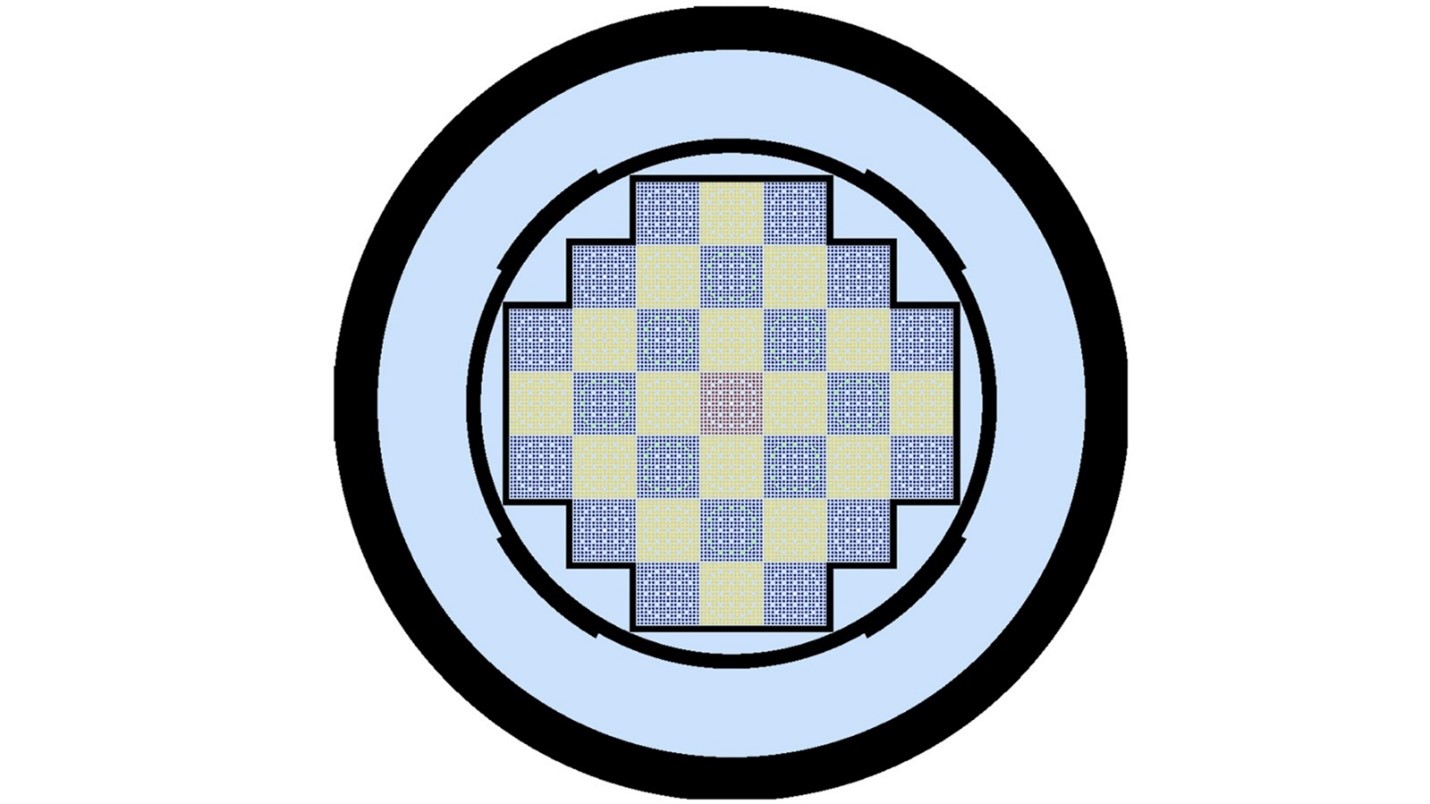
Coolant flow around the fuel pins in a light water reactor core plays a critical role in determining the reactor’s performance. For yet-to-be-built small modular reactors, a thorough understanding of coolant flow will be key to successfully designing, building, and licensing first-of-a-kind reactors.