The ongoing effort to convert the world’s research reactors
The 40-year effort to make research reactors safer and more secure has led to the conversion of 71 reactors worldwide from HEU fuel to LEU.
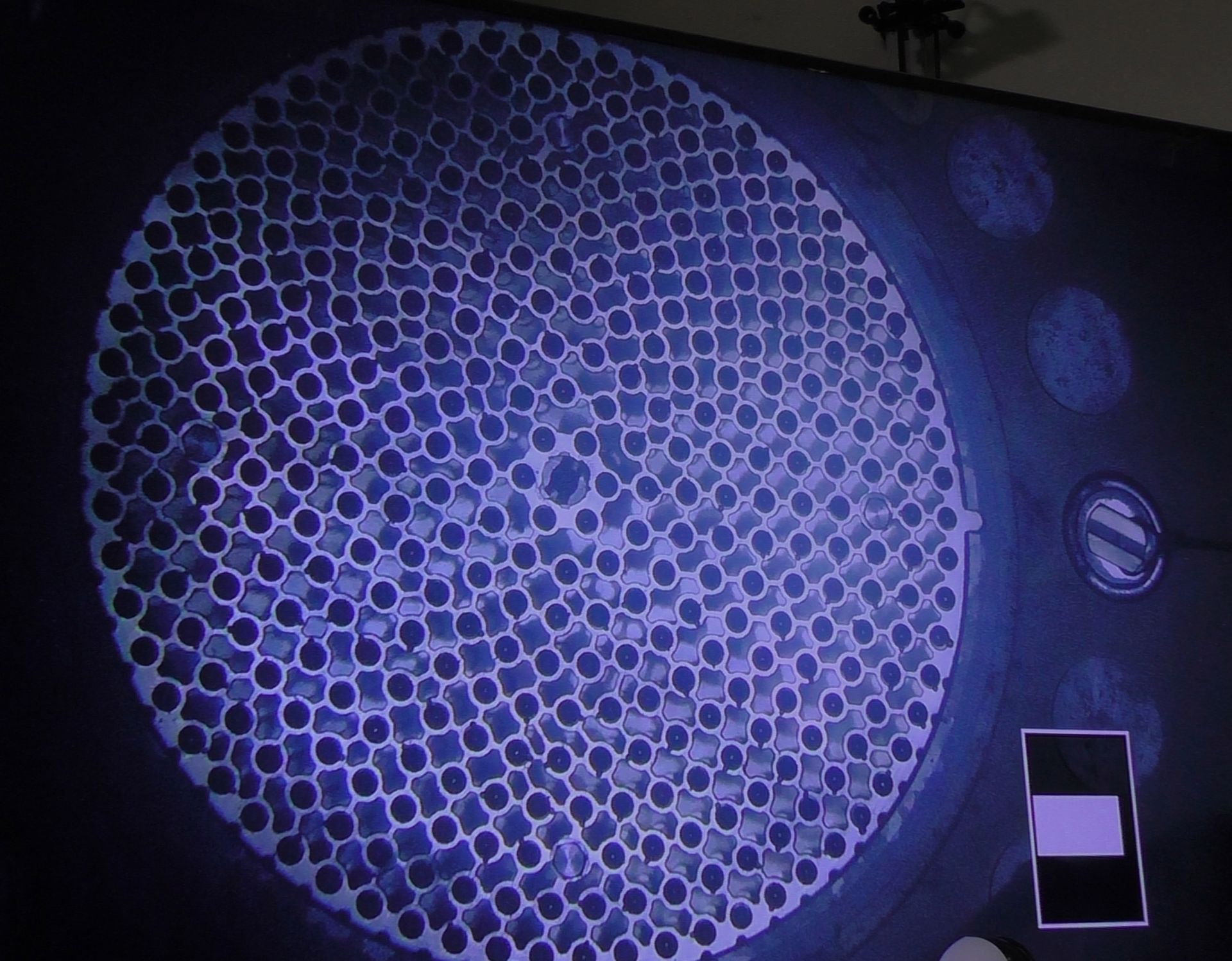
The Ghana Research Reactor-1, located in Accra, Ghana, was converted from HEU fuel to LEU in 2017. Photo: Argonne National Laboratory
In late 2018, Nigeria’s sole operating nuclear research reactor, NIRR-1, switched to a safer uranium fuel. Coming just 18 months on the heels of a celebrated conversion in Ghana, the NIRR-1 reboot passed without much fanfare. However, the switch marked an important global milestone: NIRR-1 was the last of Africa’s 11 operating research reactors to run on high-enriched uranium fuel.
The 40-year effort to make research reactors safer and more secure by replacing HEU fuel with low-enriched uranium is marked by a succession of quiet but immeasurably significant milestones like these. Before Africa, a team of engineers from many organizations, including the U.S. Department of Energy’s Argonne National Laboratory, concluded its conversion work in South America and Australia. Worldwide, 71 reactors in nearly 40 countries have undergone conversions to LEU, defined as less than 20 percent uranium-235. Another 31 research reactors have been permanently shut down.